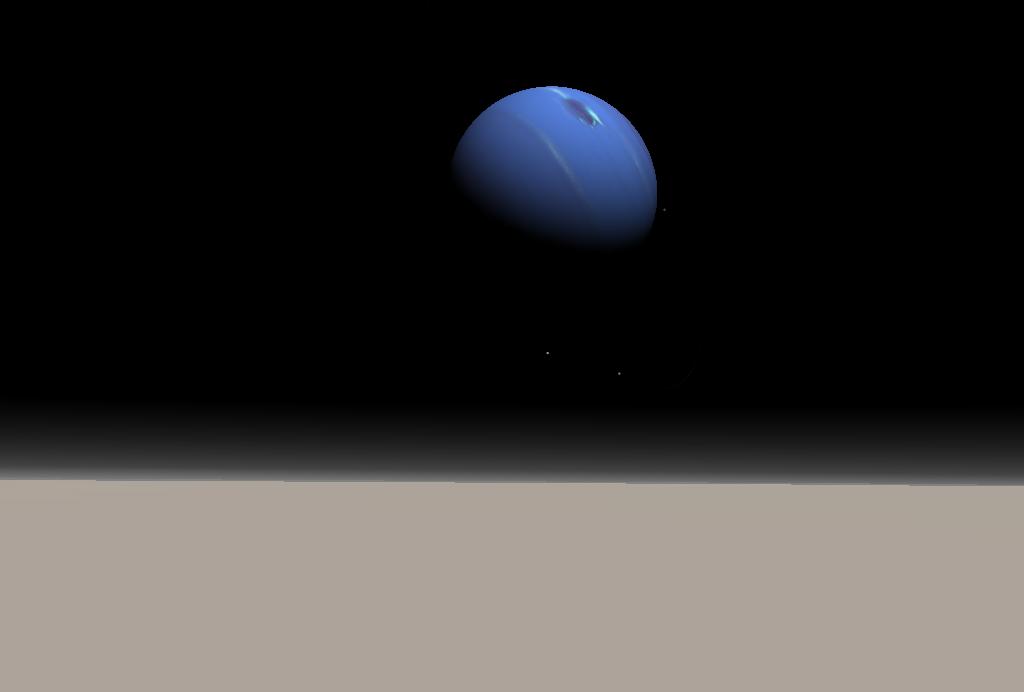
“Four happy days bring in Another moon;
but O, methinks, how slow This old moon wanes!”
— William Shakespeare, “A Midsummer Night’s Dream” (1595)
Far from the Sun, into the deep reaches of the outer Solar System, Uranus and Neptune mark the realm of the ice giants—a distinct class of planets that have a different layered internal structure from Jupiter and Saturn, largely composed of hot, dense, superfluid water, ammonia, and methane “ices.” As is the case with their gas giant cousins, Uranus and Neptune are also accompanied by their respective large assortments of moons, some of which have proven to be surprisingly geologically active, even in this cold, dark region of the outer Solar System.
The enigmatic moons of the ‘Nebulous Star’
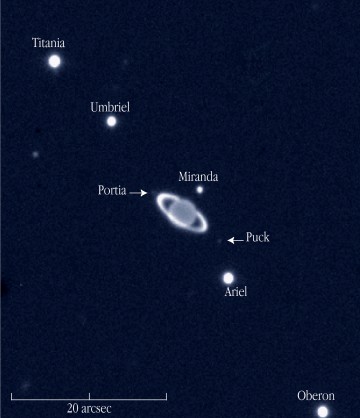
Uranus was the first planet ever to be discovered with a telescope during the 18th century, when in 1781 German-British astronomer Sir William Herschel spotted in the constellation Taurus something that he described as “a Comet or a Nebulous Star.” Even though it turned out that the same object had also been observed by various astronomers in previous times, it was Herschel’s findings which led to the realisation that the newly found comet-like object was indeed a planet orbiting the Sun beyond the then-known limits of the Solar System. It wasn’t many years later when, in 1787, Herschel would discover two of Uranus’ five largest moons as well, which were eventually named Titania and Oberon by his son John Herschel, after fictional characters from William Shakespeare’s comedy play “A Midsummer Night’s Dream.” Many decades later, in 1851, English astronomer William Lassell would find another two moons orbiting the distant planet, which in keeping with the emerging trend of naming any newly discovered objects in the Uranian system from characters in British literature, were called Ariel and Umbriel, after the names of two sky spirits in Alexander Pope’s satirical poem “The Rape of the Lock.” Almost a century would pass until famous American astronomer Gerard Kuiper would discover Miranda in 1948, the last of Uranus’ five large moons.
As was the case with every other outer Solar System planet, almost nothing was known about Uranus prior to the advent of the space age in the second half of the 20th century. The launch of NASA’s twin Voyager spacecraft in 1977 changed all that forever, by transforming the outer planets Jupiter, Saturn, Uranus, Neptune, and their moons from blurry points of light in the sky into real and fascinating worlds of unimaginable beauty. Throughout their mission, the two Voyager spacecraft also discovered dozens of new moons around these far-off worlds, which were too small and faint to be detected by ground-based observatories, revealing the outer planets and their moons in their true dimension as mini, intricate solar systems in their own right. Similarly, when Voyager 2 flew by Uranus in January 1986, it provided humanity with its first close-up views ever not only of the distant lopsided planet itself and its already known five moons, but of 10 more inconspicuous, tiny inner moonlets no more than a few dozen kilometers in diameter that had very close orbits to the planet. In the following decades, 12 additional tiny, irregular satellites in highly inclined orbits detected by the Hubble space telescope and various ground-based observatories have brought Uranus’ current moon count to 27.
Yet, even though Voyager 2’s flyby has provided us with the bulk of our current knowledge of the planet, a greater series of even more intriguing questions remain unanswered to this day about this enigmatic cyan-tinted ringed and distant world, which is located 2.8 billion km from the Sun. The same applies for Uranus’ moon system as well. All of the planet’s five larger moons—Miranda, Ariel, Umbriel, Titania, and Oberon—as revealed by Voyager’s cameras exhibit heavily cratered and relatively dark surfaces that are interlaced with geologically younger areas, which hint at an intense past geologic activity. More specifically, Miranda, the smallest of Uranus’ major moons with a diameter of just 470 km, has the most unique terrain of every other moon in the Solar System, one that features extensive fault canyons which trace the moon’s surface and are as much as 20 km deep—12 times deeper than the Grand Canyon on Earth. Furthermore, three large, white-colored structures called “coronae” also dominate the landscape—a system of bright ridges and valleys that come in sharp contrast with the moon’s darker areas. When viewed from afar, Miranda’s surface looks like a giant jig-saw puzzle whose pieces were put randomly together, producing an asymmetric and chaotic result.
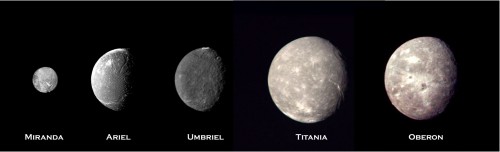
Orbiting farther out, the 1,158-km-wide moon Ariel exhibits similar surface features with Miranda, with impact craters, fault scraps, ridges, and deep, flat canyons crisscrossing the moon’s landscape. The largest of these canyons, called Kachina Chasmata, is approximately 622 km long, which is bigger than the moon’s radius. Ariel’s surface is also the brightest and the youngest of all of Uranus’ moons, which means that it must have been the most geologically active as well, something that is also derived by the evidence of past volcanic activity that can be seen throughout the moon. The same evidence can also be seen on Titania, Uranus’ bigger moon, which has a diameter of 1,576 km. Even though the latter’s surface is less cratered than that of other Uranian moons, it largest impact crater, Gertrude, is 326 km across. Other prominent features on Titania’s surface include extensive networks of interconnected canyons, the largest one of which, Messina Chasmata, is 1,492 km long, running across the moon’s surface from the equator to the south pole. By studying the images that were beamed back by Voyager 2, scientists have theorized that Titania’s entire surface must have been extensively reshaped at some point in the past, possibly due to periods of intense water-rich cryovolcanic activity which led to the young, smooth plains that are observed on the moon’s present-day surface. The same activity is believed to have also taken place on Miranda, Ariel, and Oberon as well, hinting at the possible presence of underground layers of liquid water in these moons’ interiors sometime in their history.
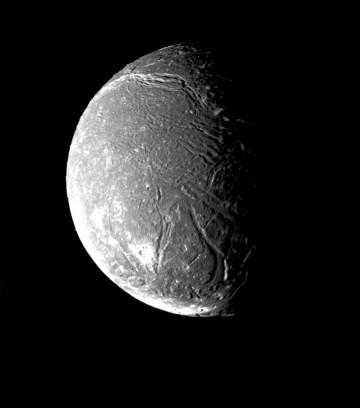
Even though Uranus’ five larger moons are equally composed of water ice and rocky materials, scientists were originally uncertain as to the potential of their internal ices being partially melted in order to form any underground layers of liquid water. Located in the cold, deep reaches of the outer Solar System these moons were seen as being too small to have retained any internal heat processes that would be sufficient enough to keep any underground liquid water reservoirs from freezing. Yet, as the exploration of the Jovian and Saturnian systems by the Galileo and Cassini spacecraft respectively have indicated, moons as small as 500 km wide in the case of Enceladus could possibly feature underground liquid water oceans in part due to tidal heating forces from their host planet’s gravity. Furthermore, a number of studies have shown that the interiors of icy moons could potentially be kept warm besides tidal heating, from the decay of radioactive isotopes in their mantle or crust as well.
One such study was published in the November 2006 issue of the journal Icarus by a research team led by Dr. Hauke Hußmann, now at the German Aerospace Center’s Institute of Planetary Research in Berlin. The researchers examined several internal structure models for various middle-sized icy moons of Saturn, Uranus, and Neptune, as well as several large trans-Neptunian objects like Pluto, Charon, Eris, and Sedna, in order to investigate under which conditions any underground liquid oceans inside these celestial objects were possible. Their results showed that in the case of Uranus, assuming a sufficient heat production from radioactive decay, the interiors of the ice giant’s two larger moons Titania and Oberon could indeed host such liquid water oceans. The latter, if present, could also be kept from freezing by the presence of other volatiles like ammonia that are abundant in the outer Solar System, which significantly lower the freezing point of water, keeping it liquid at temperatures as low as – 97 degrees Celsius. “Moons with considerable H2O and silicate contents may have harbored internal oceans up to the present time since the silicate components of those satellites were massive enough to generate heat from the decay of long-lived radiogenic isotopes at a rate sufficient to prevent the freeze-out of liquid subsurface reservoirs,” write the researchers in their study. “An important factor for the existence of liquid layers is the presence of ammonia or other volatile constituents. Even small amounts of those additional components will reduce the melting temperature of H2O significantly, making the existence of internal oceans more likely … Including initial ammonia abundances based on most recent observational evidence, we obtain [in the study’s results] subsurface oceans even at the present time for Europa, Rhea, Titania, Oberon, Triton, Pluto, and the trans-Neptunian objects 2003 UB313 (Eris), Sedna and 2004 DW (Orcus).” Even though these results are certainly intriguing, the researchers are nevertheless quick to point out that the study is based on various assumptions regarding the internal structure of icy moons that, in the absence of more observational evidence from dedicated robotic space missions to these worlds, cannot be confirmed or invalidated at this point. “In general, we argue that internal oceans may be a common phenomenon (at least in the past) of the satellites of the outer Solar System and other icy bodies like the large trans-Neptunian objects,” they conclude in their study. “The consequences of subsurface oceans are important for these planetary bodies with respect to orbital evolution, internal dynamics, and astrobiological implications. However, more observations are required to confirm or disprove their existence.”
Underground oceans near the planetary god of the sea?
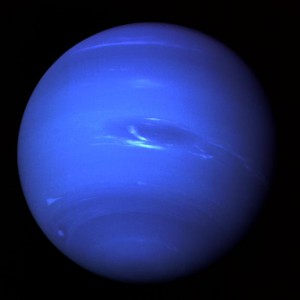
Even though Italian astronomer Galileo Galilei first spotted Neptune with his telescope in 1612, he didn’t recognise it as a planet, but had mistakenly perceived it as a fixed star in the sky due to its slow retrograde motion at the time. The realisation of the beautiful, blue-tinted world’s real nature would have to wait until the 1840s. In their attempts to explain a series of observed perturbations in Uranus’ orbit, which had been discovered several decades earlier, British astronomer John Adams and French mathematician Urbain Le Verrier both independently developed calculations of their own during that time, which showed that the cause of the observed perturbations in Uranus’ orbit were due to the gravitational tug of another, as yet unknown planet. Based on Le Verrier’s calculations, German astronomer Johann Galle succeeded in spotting the hypothesized planet close to the predicted position in the sky in September 1846 using the 9.6-inch refracting telescope of the-then new Royal Observatory in Berlin, thus making Neptune the first planet whose existence was confirmed by telescopic observations after it had been discovered on paper first. It was just 17 days later that the new-found planet’s biggest satellite, eventually named Triton, was discovered as well by English astronomer William Lassell. The rest of the gas giant’s 14 known moons are irregular tiny moonlets with diameters that range from a few dozen to a few hundred kilometers and were gradually discovered in the following centuries. The second and third satellites of Neptune were found in 1949 and 1981 respectively, while the Voyager 2 spacecraft discovered another six during its close flyby the planet 25 years ago, in August 1989. The remaining five were spotted in the years following Voyager’s Neptune encounter.
Neptune was the last planet to be visited by Voyager 2, representing the culmination of the latter’s 12-year Grand Tour of the outer Solar System. As detailed in a recent history series by AmericaSpace writer Ben Evans which commemorated the event’s 25th anniversary, Neptune put on a quite a show for Voyager 2, revealing a surprisingly active and dynamic atmosphere and weather patterns to the spacecraft’s instruments, despite its distance of 4.5 billion kilometers from the Sun. Because the planet radiates more than twice the energy that it receives from the distant Sun, it drives the fastest winds seen in the Solar System, reaching speeds as high as 2,400 km per hour. And contrary to Uranus’ “bland” and “boring” atmosphere, that of Neptune was full of dramatic features like streaks of white high-altitude clouds racing around the planet and two Great Dark Spots, large elliptically shaped anti-cyclonic storms that eventually dissipated and disappeared in the years following the Voyager flyby. Yet what stole the show was Triton, Neptune’s 2,700-km-wide moon. Even though Triton was measured to have one of the coldest surfaces ever in the entire Solar System (just 38 Kelvin above absolute zero), it was nevertheless found to exhibit a surprising geologic activity. When Voyager 2 flew over the moon’s northern hemisphere a day after the Neptune encounter, it transmitted back to the unsuspecting scientists on Earth images of geyser-like eruptions of gaseous nitrogen coming from an almost crater-free and geologically young surface, which formed plumes up to 8 km high into Triton’s tenuous atmosphere. This sight of active cryovolcanism on a moon located 4.5 billion km from the Sun was what led many members of the Voyager team at the time to jubilantly exclaim “Wow! What a way to leave the Solar System!”

A strange and unique characteristic of Triton is that it orbits Neptune in a direction that is opposite to the planet’s rotation. Scientists had long hypothesized that this peculiar retrograde motion is evidence that the moon didn’t form around Neptune, but originated somewhere in the Kuiper Belt instead and was later captured by the massive planet’s gravity in an originally highly elliptical orbit before eventually settling into its present almost circular one. According to theoretical models, this gradual reshaping of Triton’s orbit would have raised large tides on the moon’s surface, while the tidal stress from Neptune would have melted the moon’s internal ice layers, which in turn would have formed large underground oceans of liquid water. Could this underground water reservoir have remained liquid to the present day?
According to a study published in the August 2012 issue of the journal Icarus by a team led by Jodi Gaeman, a geophysicist from the University of Maryland’s Department of Geology, if Triton’s orbit around Neptune was an originally highly elliptical one which circularized over time, then the dissipation of the tidal energy from Neptune’s immense gravity coupled with the heat from Triton’s radioactive decay would keep the moon’s underground ocean liquid for long periods of time up to the present. As with Uranus’ large moons, the researchers believe that Triton’s ocean is also rich in ammonia, something that would retain the ocean’s water liquid at much lower temperatures. “I think it is extremely likely that a subsurface ammonia-rich ocean exists in Triton,” said Dr. Saswata Hier-Majumder, now a Senior Lecturer at the Royal Holloway University of London and co-author of the study, in an interview for the Astrobiology magazine. “[But] there are a number of uncertainties in our knowledge of Triton’s interior and past which makes it difficult to predict with absolute certainty.”
As is the case with Uranus, the lack of more detailed observations of the Neptune system that could come from a dedicated space probe to the distant realm of the ice giants in the outer Solar System forbids us from gaining a deeper understanding of the processes that have driven the evolution of Triton and its hypothesized underground ocean. Until the day comes when new space missions set sail for mystifying Uranus, Neptune, and their moons, the notion of Triton’s underground waters will just remain in the realm of intriguing, but ultimately unconfirmed speculation.
Infinite diversity in infinite combinations?
Astrobiology is the science that studies the origin and evolution of life in the Universe. Quite naturally, with only one example of life—that on Earth—this field of scientific research tries to determine if similar types of life (based on the presence of organic compounds, liquid water, and energy) also could have developed in other parts of the Solar System and beyond. As this article series has shown, microbial life on Earth can develop almost in any place imaginable under extreme conditions that would be detrimental to every other terrestrial life forms, as was showcased by the recent findings of an entire microbial ecosystem thriving in the frigid subglacial Lake Whillans in Western Antarctica. “Life on Earth can exist in many extreme environments and it can do many remarkable things and we’re learning more every day, about how flexible and remarkable life on Earth really is,” says Chris McKay, a senior scientist at the Space Science and Astrobiology Division at NASA’s Ames Research Center in California. In addition, the extraterrestrial environments of many icy moons in the outer Solar System seem capable of providing similar conditions for supporting the emergence life as we know it. One of these moons, Titan, holds the promise of life which, if present, would have a very different biochemistry than that on Earth, one which would be based on liquid methane instead of liquid water. “To me the most exciting possibility is that there’s life on Titan, because that would show not just that life started twice [in the Solar System], but it started twice in very different conditions,” adds McKay. “It would show us that life is a natural process that it’s gonna pop up on many different worlds, on many different planets around many different stars.”

But in our search for life in the Universe, a more fundamental question arises: What is life? Scientists, philosophers, and theologians alike have tried hard for millenia to provide a satisfactory answer to this simple question, to no avail. Yet, even though a comprehensive definition of life eludes us, science can describe some basic properties that define all living things: the ability to organize simpler chemical substances into more complex ones, the use of available energy to drive their metabolic processes, the ability to grow and reproduce, and the ability to respond and adapt to their environment. Nevertheless, many organised systems can mimic several of these properties without themselves being considered living organisms, further perplexing our quest for a definition of life. For instance, fire, which is the result of the chemical process of combustion between a fuel and an oxidant, can use the energy released to grow and reproduce without being considered alive.
Furthermore, the results of a study, published by an international research team in the August 2007 issue of the New Journal of Physics, demonstrated using a computer model of molecular dynamics that organized collections of inorganic plasma crystals, found to be prevalent throughout the Universe in places like the interstellar medium, the tails of comets, and the rings of Saturn, could under the right conditions develop complex helical structures that resembled that of DNA, while also showcasing the ability to evolve and divide in order to replicate themselves. Could these inorganic structures also be developed naturally in the harsh conditions of space? Could they also be considered a form of life? The researchers reach a startling conclusion: “Our analysis shows that if helical dust [plasma] structures are formed in space, they can have bifurcations as memory marks and duplicate each other, and they would reveal a faster evolution rate by competing for ‘food’ (surrounding plasma fluxes). These structures can have all necessary features to form ‘inorganic life’. This should be taken into account for formulation of a new SETI-like program based not only on astrophysical observations but also on planned new laboratory experiments, including those on the International Space Station. In the case of the success of such a program one should be faced with the possibility of resolving the low rate of evolution of organic life by investigating the possibility that the inorganic life ‘invents’ the organic life.”
The results of this study reminds us that we should always keep an open mind in our search for life in the Universe, while critically examining every finding at the same time, even if it seems counter-intuitive. “We really have to put ourselves out there, in terms of thinking what the possibilities are,” says Dr. Jim Green, director of NASA’s Planetary Science Division. [What we find] might be extreme life, it might be life that we have never seen before in terms of its structure and its composition.”
It is in this manner that we can hope to one day make the first such definite discovery.
Video Credit: ESA
Make sure to read Part 1, Part 2, Part 3, and Part 4.
References:
1. “Finding Life Beyond Earth,” PBS, 2012
2. “Uranus, Neptune, Pluto, and the Outer Solar System, Revised Edition”, by Linda T. Elkins-Tanton, Facts On File, Inc, Infobase Publishing (2011)
3. “Life beyond Earth – The Search for Habitable Worlds in The Universe”, by Athena Coustenis and Thérèse Encrenaz, Cambridge University Press (2013)
4. “‘What a way to leave the solar system.’ (discoveries from Voyager 2).”, by Eberhart, J., Science News, Sep. 2, 1989 (former Science Service, Inc). Retrieved from The Free Library.
5. http://www.sciencedirect.com/science/article/pii/S0019103512001790
6. http://www.sciencedirect.com/science/article/pii/S0019103512001790
7. http://www.astrobio.net/news-exclusive/does-triton-have-a-subsurface-ocean/
8. http://iopscience.iop.org/1367-2630/9/8/263
Want to keep up-to-date with all things space? Be sure to “Like” AmericaSpace on Facebook and follow us on Twitter: @AmericaSpace
Makes one wonder what life would be like today if we’d gone to Saturn in the 70s with project Orion on a ship and staff the size of an ocean liner?
If that prosperous future had come to pass, we might be scheduling right now our vacation trips to witness Saturn’s rings up close and ice skate near Enceladus’ ‘tiger stripes’.
Too bad the gates of the final frontier were eventually closed back then, just as we were about to open them up. I hope we can do better next time.
Great article! Another essay published today on habitable moons can be found at the following:
http://www.drewexmachina.com/2014/09/19/habitable-moons-background-and-prospects/
Thank you Mr. LePage!
I’m also a regular reader of your blog, which I find to be a great source of historical information and analysis.
Kind regards.
And thank you, Mr. Papadopoulos for your kind words about my blog.
http://www.drewexmachina.com/blog/
Fascinating and important article that continues to expand our knowledge and understanding of the gas giants.
Could it be that gas giants, by definition, evolve into mini solars systems (with multiple moons) throughout the universe?
There’s no reason Tom, to think that exoplanets could not form their own moon systems around other stars. As past systematic observations during the last decades have shown, planetary formation is a process of accretion of material inside protoplanetary disks and we have observed the latter throughout the galaxy.
Even though exomoons are beyond the observing capability of today’s instruments, the next generation of ground and space-based telescopes that will come online in the next few years should be able to spot them if they are there, thus opening up a new research field on exoplanetary science. Indeed, exciting times ahead!
Leonidas, thank you for your response. Makes perfect sense and the next generation telescopes can’t come soon enough.