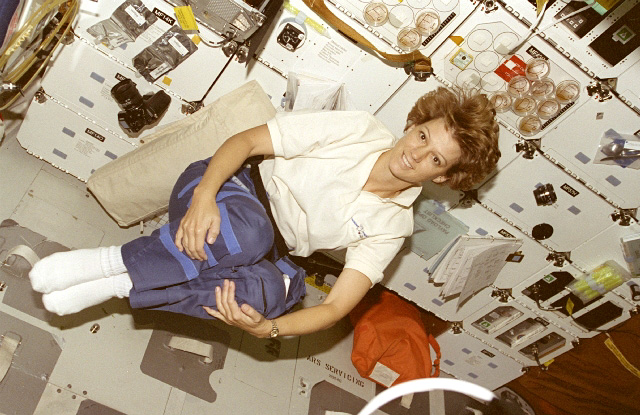
“It’s great to be back in zero-g again,” said STS-93 commander Eileen Collins, early on 23 July 1999, as she and her four crewmates set about preparing the Shuttle Columbia for five days of orbital activities, but added darkly that “a few things to work on ascent kept it interesting.” Those things, within seconds of liftoff, almost forced Collins—the first woman to command a space mission, 15 years ago, this week—to perform a hair-raising abort landing back at the Shuttle Landing Facility (SLF) at the Kennedy Space Center (KSC), and the incident effectively grounded the shuttle fleet for almost six months.
Columbia’s flight was a long time coming. More than a year had elapsed since her last mission, but the cause of the delay had nothing to do with NASA’s venerable old workhorse herself. Originally scheduled for launch in August 1998, it was postponed until December, then January 1999, then April, and ultimately midsummer, by a chain of technical problems with STS-93’s primary payload, the $1.5 billion Chandra X-ray Observatory and the Boeing-built Inertial Upper Stage (IUS) which would propel it into an unusual orbit. Columbia—the oldest member of the fleet of shuttle orbiters and the only one not yet to have been modified with an International Space Station (ISS)-specification airlock and docking mechanism in her payload bay—was ideally tasked to deliver Chandra into space.
The reason for this was Chandra’s sheet size. When affixed to the IUS and mounted on a supporting “tilt table,” the observatory consumed 57 feet (17.4 meters) of Columbia’s 60-foot-long (18.3-meter) payload bay and weighed more than 49,800 pounds (22,600 kg). In anticipation of their back-to-back ISS flights, the other three orbiters had already had their internal airlocks removed from the middeck and replaced inside the docking mechanism in the forward quarter of their payload bays. The result was that there was not enough room in the bays of Discovery, Atlantis, or Endeavour to house Chandra. Yet even Columbia herself had to lose 7,000 pounds (3,200 kg) of additional mass before she could transport the new observatory aloft. To achieve some of these savings, engineers used older, lighter main engines, which lacked the newer and more rugged high-speed fuel pumps and combustion chambers. “We put Columbia on a strict diet to get to this mission,” said processing manager Grant Cates. “That work actually began [in 1996] with the identification of this mission and the weight reduction that would be required.” Nonetheless, the orbiter’s weight would still creep above NASA’s normal safety limit in the event that an emergency landing was required, shortly after launch.
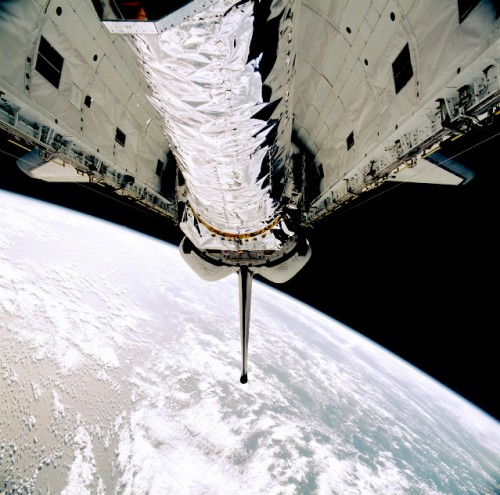
In such an eventuality, Columbia would tip the scales at 249,000 pounds (113,000 kg), some 1,300 pounds (590 kg) heavier than safety rules mandated as the maximum allowable landing weight. In the case of STS-93, a one-time-only waiver was granted to this rule, based on a detailed analysis of the payload, the shuttle’s center-of-gravity constraints, and a host of interrelated factors. For Eileen Collins, the challenge of possibly having to perform a heavier-than-normal emergency landing, known as a Return to Launch Site (RTLS), did not faze her. “We would land at 205 knots, which is very close to the maximum certification around 214,” she explained in a pre-launch interview. “There are some challenges there, but I feel very confident we’ve looked at the abort landings and they’re well within the safe limits of landing the shuttle.”
Collins’ confidence in her abilities and those of her crew would come close to being tested. Joining her and “rookie” pilot Jeff Ashby were mission specialists Catherine “Cady” Coleman, Steve Hawley, and Frenchman Michel Tognini. In November 1997, Tognini became the first member of the STS-93 crew to be announced by NASA and was joined by Collins, Ashby, Coleman, and Hawley in March 1998. “I was very happy because I had been waiting for such a long time to get this assignment,” Tognini told a NASA interviewer. “At one point, I was expected to have a flight on shuttle-Mir, because I spoke Russian and I flew previously on Mir and Soyuz. Instead of flying to Mir, they asked me not only to fly on STS-93, but to also be in charge of the deployment of Chandra with Cady Coleman. I was very surprised and proud to have such a challenge and such responsibility.”
Making his fifth shuttle, Steve Hawley had been newly appointed as deputy director of the Flight Crew Operations Directorate at the Johnson Space Center (JSC) in Houston, Texas, when he was approached to join the STS-93 crew. “I think they were looking for an experienced person for that mission,” he told the NASA oral historian. “It was a relatively junior crew. I think they were looking for somebody experienced to add to the mix of relatively less experienced people.” When he was first asked, Hawley declined the invitation to join the crew, having only recently flown aboard the STS-82 Hubble Space Telescope servicing mission. “I thought I really hadn’t been back in my real job that long yet,” he explained to the oral historian, “and I didn’t think that it was appropriate for me to step aside and fly again that soon, but they pestered me and so I did it, but I honestly told them that I didn’t think it was the right thing to do.”
In many ways, STS-93 harked back to the early days of the shuttle program. Not only would it last just five days—making it NASA’s shortest planned mission for almost eight years—but it would also feature the deployment of the orbiter’s final IUS payload. Built by Boeing and provided by the U.S. Air Force, the IUS had originally been developed as a short-term stand-in for a reusable “space tug” and was initially dubbed the “Interim Upper Stage,” before changing to “Inertial” in recognition of its sophisticated internal guidance system. Its first shuttle use on STS-6 in April 1983 put a key NASA communications satellite into a lower-than-planned orbit. This failure led to several missions being postponed, but the IUS eventually established itself as an exceptionally reliable booster.
However, it was still prone to difficulties. On 9 April 1999, a Titan IV rocket carried a Defense Support Program (DSP) missile early-warning satellite aloft, but both the first and second stages of the IUS failed to separate properly and the $250 million payload was lost. Ordinarily, NASA would have watched the resulting investigation with interest and incorporated its findings into its own plans to ready Chandra’s IUS for launch. This was complicated, however, by the fact that the investigation itself was classified. Moreover, said Scott Higginbotham, who oversaw Chandra’s pre-flight processing at KSC, the IUS assigned to STS-93 was impounded as part of the investigation. Original plans had called for it to be attached to Chandra on 23 April, but this was delayed as investigators set to work. Columbia’s launch, then scheduled for 9 July, came under review and the impounded IUS had a domino-like effect on the training of the astronauts and their ground teams.
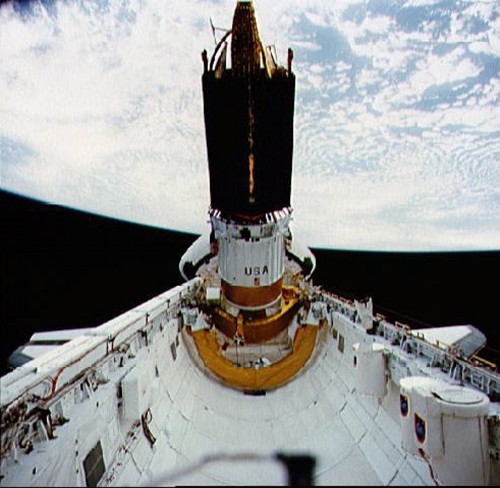
“We were planning a two-day-long sim, starting 14 April, that would involve all the different control centers, a joint integrated simulation, with everybody,” explained STS-93 Lead Flight Director Bryan Austin. “That was going to be a big deal. That has been postponed because the Air Force folks and Boeing IUS people were going to be taken away initially to be part of the investigation. That exercise has been put on hold. That kind of put a wrench in things in terms of our sim schedule.”
The launch of Chandra was also significant because it was the shuttle’s first IUS mission since STS-70 in July 1995, and, according to Austin, “there has been a lot of change in the expertise level, collectively. For the most part, IUS deployment procedures are the same, but something that, to me, has been a struggle for this flight with some of our IUS friends is to get them to realize that the payload on the other end of the IUS is not the typical thing that the shuttle has been doing.” His point was that, as soon as the Chandra/IUS combo transitioned to internal battery power, just minutes before deployment, the observatory would be on its own. On previous missions, if something went awry at the last moment, the IUS and its payload could be retracted for another attempt or returned to Earth. In the case of Chandra, power and temperature constraints meant that mission managers had just a single shot at a deployment. “It’s either going to become orbiting space trash,” said Austin, grimly, “or it’s going to go out and do its mission.”
In fact, Chandra was the third in a quartet of “Great Observatories” which NASA had been planning for more than two decades to explore the Universe with sensors that jointly covered virtually the entire electromagnetic spectrum. The first two observatories—Hubble, launched in April 1990, and the Compton Gamma Ray Observatory, which followed in April 1991—focused on visible and ultraviolet wavelengths, as well as high-energy gamma radiation. Two others would then cover X-rays (Chandra) and infrared (the 2003-launched Spitzer Space Telescope). “Hubble revealed the visible side of the Universe,” said theorist Michael Turner of the University of Chicago in Chicago, Ill., “but most of the Universe does not emit visible light. It’s only visible by other means, in particular the X-rays. Chandra will give us the same clarity of vision as Hubble does, but for the ‘dark’ side of the Universe we know the least about.” Comparing astronomers’ capabilities before and after Chandra, astronaut Steve Hawley likened it to the difference between the small reflecting telescope he had used as a child and the enormous observatory on Mount Palomar in San Diego, Calif.
“We can make Superman jealous with our X-ray vision!” claimed Ken Ledbetter, NASA’s head of mission development for Chandra. The observatory, which received Congressional approval in 1987, used the most precisely figured X-ray mirrors ever built. It was hoped to equal, or even surpass, Hubble by studying some of the most exotic phenomena in the known Universe, including quasars, black holes, and white dwarfs. The spacecraft was a tapering cylinder, 45.3 feet (13.8 meters) in length, with two solar arrays at its base to provide electrical power. At the opposite end, mounted in the telescope’s primary focus, were its two scientific instruments: the High Resolution Camera and the CCD Imaging Spectrometer. Chandra’s concentric nest of cylindrical mirrors were coated with reflective iridium and gave it ten times the resolution of existing X-ray detectors and 50 times the sensitivity.
Of the many astronomical targets for Chandra, black holes were sure to seize the public’s interest, even though so little was known about them. By definition, they are “unobservable,” but the capability existed to indirectly study them by analysing radiation emitted by material being sucked into them. As interstellar gas and dust is accelerated, for example, it collides with increased energy levels and emits X-rays before vanishing across the “event horizon.” By examining such emissions in unprecedented detail with Chandra, astrophysicists hoped to identify black hole signatures. Additionally, supernova explosions thought to lead to black holes were placed under scrutiny. One of the observatory’s first celestial targets was the remnant of a massive star in the Large Magellanic Cloud that was seen to explode in 1989. On a far larger scale, it was anticipated that Chandra would focus on the amount of dark matter present in the Universe, by carefully examining galactic clusters. Such galaxies which make up these clusters are deeply embedded in huge clouds of hot, X-ray-emitting gas and are held in place by gravity generated by all the components of the cluster. Its observations were thus expected to enable astrophysicists to refine their numbers of how much “normal” matter is present in a given cluster and thus how much “dark” matter must also be present in order to generate gravity needed to hold it together.
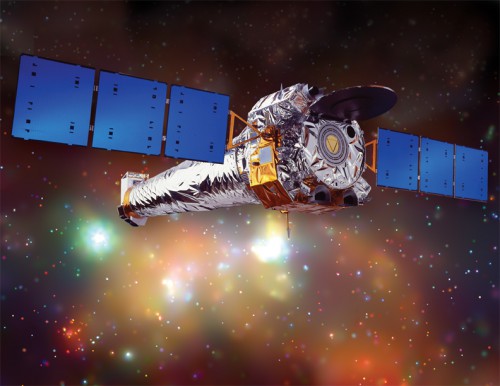
Chandra was originally conceived in the 1970s, when NASA envisaged a Large Orbiting X-ray Telescope. That was later de-scoped and became the HEAO-2 Einstein Observatory, whose success prompted the space agency to include an X-ray telescope on its wish list for a four-spacecraft flotilla of Great Observatories. Early plans called for it to be launched into low-Earth orbit and periodically serviced by shuttle spacewalkers for a projected 15-year lifespan, but in 1991 escalating costs and technical problems forced a rethink of the mission. It was effectively split into two halves. One half would transport a high-resolution camera and imaging spectrometer into a highly elliptical orbit, which, although beyond the reach of the shuttle, would enable it to gather data from 55 hours of each 64-hour orbit. Meanwhile, the second half would be equipped with a super-cooled X-ray spectrometer and would be launched into a lower orbit, but was cancelled by NASA in 1993 in another round of budget cuts. Even with the cancellation of the second mission, Chandra—known at the time as the Advanced X-ray Astrophysics Facility (AXAF)—was still expected to cost $3 billion during its first eight years of operations, including the spacecraft itself, the shuttle and IUS launch costs, annual mission control and data analysis fees, use of the Tracking and Data Relay Satellite System (TDRSS), and a one-time charge to test the mirrors at NASA’s X-ray Calibration Facility (XRCF) at the Marshall Space Flight Center in Huntsville, Ala.
Nevertheless, even this cost was far cheaper than the $7 billion envisaged for an all-in-one AXAF in low-Earth orbit. The observatory’s two largest mirrors—each measuring 48 inches (122 cm) in diameter—for its High Resolution Mirror Assembly were completed in June 1991 and tested at the XRCF in September of the same year. By January 1995, all eight nested mirrors had been completed, polished, and measured. “The first mirror took nine lengthy polishing cycles to complete,” said AXAF Telescope Project Manager John Humphreys. “We then applied a process of continual improvements to get the job done much faster and were able to complete the final mirror in only three polishing cycles.” The reflective chromium-iridium coating was applied in May 1996.
The decision to launch Chandra into a location far from shuttle repair crews was a tough sell, particularly in the wake of Hubble’s spherical aberration problems in the early 1990s. Still, the highly elliptical orbit, ranging between 6,200 miles (10,000 km) and 87,000 miles (140,000 km) from Earth, offered several advantages. It was thermally benign, eliminating the problematic cycle of light-to-dark and warm-to-cold, every 90 minutes, and thus removed the problem of temperature cycling, which tended to wear out electrical and mechanical subsystems. Additionally, the observatory would spend 85 percent of its time above Earth’s radiation belts, allowing it a large, uninterrupted portion of each orbit for celestial observations and avoiding interference from energetic particles which might otherwise overwhelm its sensitive instruments.
As with the other Great Observatories, it was always intended that Chandra would be named after an eminent astrophysicist, whose research had helped to pave the way for the work it would perform. Chandra honoured the Indian-born scientist Subramanyan Chandrasekhar (1910-1995), affectively nicknamed “Chandra,” who has been widely labeled as the father of modern astrophysics. A Nobel Prize winner for his contributions to astronomy, he also conducted valuable theoretical work on stellar evolution that established a basis for the existence of neutron stars and black holes—the very objects that his mechanised namesake would observe from its high orbit. “Chandra thought black holes were the most beautiful things in the Universe,” said Lalitha Chandrasekhar, his 88-year-old widow, who attended the observatory’s launch on STS-93. “I hear a lot of people say they are bizarre, they are exotic, but to Chandra they were just beautiful.”
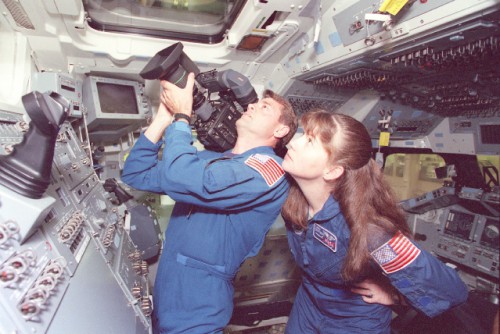
It was not, however, only the redesign of the observatory and the troublesome IUS which kept the mechanized Chandra on the ground, but also technical glitches with the spacecraft itself. Problems completing its construction at prime contractor TRW’s Redondo Beach, Calif., facility had pushed the launch date from August to December 1998, then January 1999, and ultimately April owing to a number of computer software errors. On 20 January, only days before the spacecraft was due to be shipped from California to Florida, NASA announced yet another delay, caused by the need for TRW to evaluate and correct a potential problem with several printed circuit boards in the command and data-management system. A number of other, TRW-built satellites had turned up faulty copper circuitry, and, fully aware of Chandra’s high-priority status and the fact that its orbit would render it irreparable, the decision was taken to remove and replace the boards.
Although the replacement process delayed the spacecraft’s delivery to Florida by only a matter of days, it pushed STS-93’s launch back by five weeks to late May 1999, due to the requirement to conduct lengthy tests at KSC. This target, however, conflicted with shuttle mission STS-96, the first ISS docking flight, and the decision was taken to postpone STS-93 until early July. Then, with only two weeks to go, Chandra engineers were alerted to yet another problem, this time with 20 electrical capacitors.
Fortunately, these were cleared for flight, but it offered another heart-stopping moment in the observatory’s tumultuous development. The heart-stopping moments would not end there. Before Chandra made it to orbit, STS-93 would endure one of the most hair-raising launches in shuttle history.
The second part of this article will appear tomorrow.
Want to keep up-to-date with all things space? Be sure to “Like” AmericaSpace on Facebook and follow us on Twitter: @AmericaSpace